The eleven crucial net zero technologies
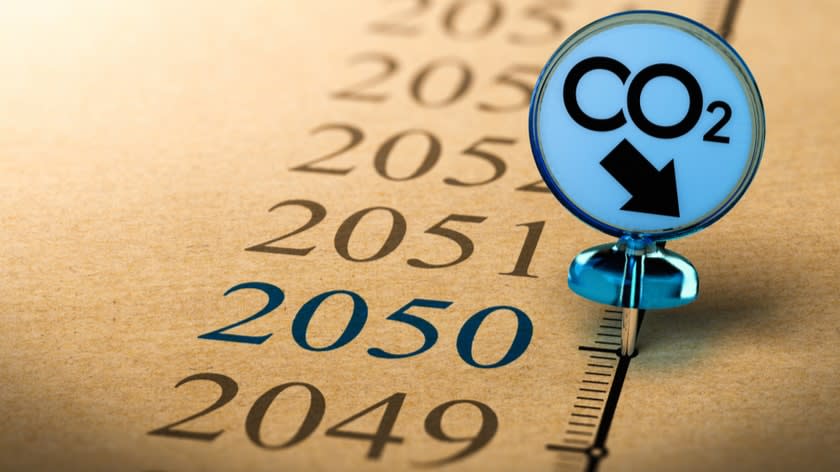
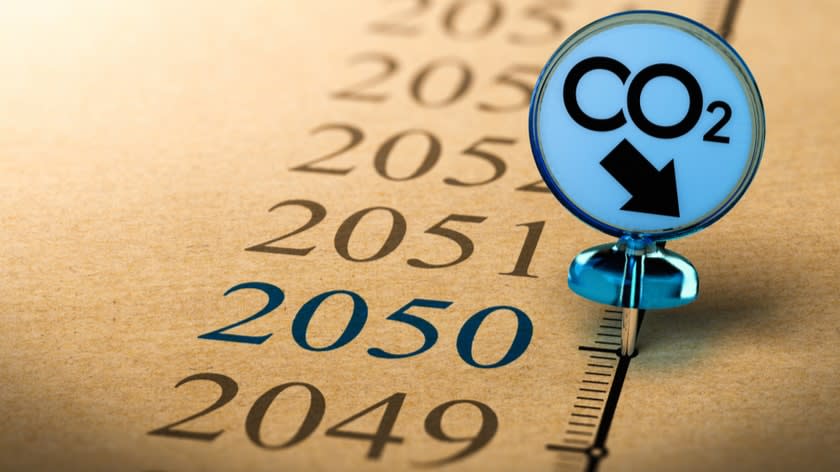
We have defined a list of eleven key technologies to help to decarbonize the world by 2050. We explain each of the technologies and what they are used for. As technologies are continuously evolving because of limitations and challenges this list is not complete and will also change over time.
We have defined a list of eleven key technologies to help to decarbonize the world by 2050
Below we explain each of the technologies and what they are used for
As technologies are continuously evolving because of limitations and challenges this list is not complete and will also change over time
Introduction
What is technology? Technology is the application of scientific knowledge to the practical aims of human life or to the change and manipulation of the human environment. Technology is constantly evolving and innovating driven by curiosity, creativity and problem-solving. Technology can be classified into different types such as electrical, digital, nanotechnology depending on the tools and methods used. Technology has enabled humans to achieve remarkable feats. And technology is key to decarbonize the world. In this report we identify eleven technologies that look set to be crucial if the world is to achieve net zero by 2050. This is a list of important technologies today and it is unlikely complete. As technology is constantly evolving and innovating, the list of today could differ substantially from this in five or ten years’ time. The important technologies mentioned below will by themselves also trigger new technologies. This is because the current crucial technologies also have challenges such as shortage of critical metals.
Crucial technologies to bring down emissions
We have defined 11 technologies/groups of technologies that are currently crucial in the transition to a net-zero world. The table below there is an overview of these. For each technology, we indicate how it is or could be used. For example a heat pump is used in the heating of homes but also in electric vehicles.
Lithium-ion batteries
A lithium-ion battery is a family of rechargeable battery types. During a discharge cycle, lithium atoms in the anode are ionized and separated from their electrons. The lithium ions move from the anode and pass through the electrolyte until they reach the cathode, where they recombine with their electrons and electrically neutralise. Lithium-ion batteries are used in vehicles, portable electrical devices and in storage. The battery's capacity and voltage are determined based on what type of active material is used in the cathode. There is a drive to increase the energy density of the battery. This is the measure of how much energy a battery contains in proportion to its weight. It is typically presented in Watt-hours per kilogram. A higher energy density means that vehicles can travel further without the need to recharge. Safety and durability are also important to take into account. Each battery chemistry has a specific energy density, stability, safety and durability and one chemistry could be better suited for storage than for electric vehicle.
Heat Pumps
A heat pump works like a refrigerator. It uses electricity to extract heat from the air, the ground, or from water, amplifies that heat, and then transfers heat to where it is needed, which in the case of a home is for space heating and hot water. Homes can install a heat pump but many energy-inefficient homes will also have to invest in energy efficiencies such as double-glazing, wall cavity insulation, and even new radiators. The primary function of heat pumps is space heating through radiators, underfloor heating systems, or warm air convectors, they can also be used to heat water for use in a home or business. In an electric vehicle, a heat pump can be used to both warm the battery and cool it. When cooling it, the excess heat from the battery can be sent to the cabin heater.
Permanent magnets
Permanent magnets are magnets that can maintain their magnetism for a long time. Permanent means that the magnet is rotating even with no electrical current applied, magnetic flux will still be present. Magnets have a high efficiency. Neodymium is the primary Rare Earth Element present in these magnets in an alloy of Neodymium-Iron-Boron (NdFeB). The alloy is then doped with other rare earth elements such as Praseodymium and Dysprosium to improve the operating characteristics of the magnet like to increase the operating temperature of the magnet without becoming demagnetised. The majority of electric vehicles have these permanent magnets in their motors. In addition, the rotating shaft of a wind turbine is connected to one or more strong magnets, usually neodymium magnets. These magnets turn relative to an assembly of coiled wire, generating voltage in the coil. Next to the neodymium magnets there are also other types of permanent magnets. Permanent magnets are used in hard drives, motors, cars, generators, televisions, phones, headphones, speakers, transducers and sensors.
Electrolysis & fuel cells
Electrolysis is a technique that uses a direct electric current (DC) to drive an otherwise non-spontaneous chemical reaction. Electrolysis is the process of using electricity to split water into hydrogen and oxygen. This reaction takes place in a unit called an electrolyser. Electrolysis is a technique and a fuel cell is a cell producing an electric current direct from a chemical reaction. Fuel cells work like batteries, but they do not run down or need recharging. They produce electricity and heat as long as fuel (such as hydrogen) is supplied. Fuel cells are unique in terms of the variety of their potential applications; they can use a wide range of fuels and feedstocks and can provide power for systems as large as a utility power station, a car and as small as a laptop computer. A fuel cell consists of two electrodes—a negative electrode (or anode) and a positive electrode (or cathode)—sandwiched around an electrolyte. In a hydrogen fuel cell, a catalyst at the anode separates hydrogen molecules into protons and electrons, which take different paths to the cathode. The electrons go through an external circuit, creating a flow of electricity. The protons migrate through the electrolyte to the cathode, where they unite with oxygen and the electrons to produce water and heat (source ). There are different types of fuel cells and every type has its own application. We would like to highlight one type though. This is the polymer electrolyte membrane fuel cell or proton exchange membrane fuel cell. In vehicles this fuel cell is mostly used. The fuel is hydrogen and operates at relatively low temperatures.
Photovoltaics (PV)
Photovoltaics is the science of turning light into electricity using special materials that absorb photons and release electrons. A photovoltaic system consists of solar panels, which are made of many connected solar cells, and a solar inverter, which converts the direct current into alternating current for use. Photovoltaic technologies vary in the type and efficiency of the semiconducting materials used, such as silicon, thin film, or organic compounds. Solar-cell efficiency refers to the portion of energy in the form of sunlight that can be converted via into electricity by the . Solar panels efficiency levels are relatively low (between 14%-25%), most home solar panels have efficiency ratings between 19% and 21% (source: ). For a continuous supply of electric power, especially for on-grid connections, photovoltaic panels require not only inverters but also storage batteries.
Concentrated solar power (CSP)
Concentrated solar power (CSP) is an approach to generating electricity through mirrors. This technology uses mirrors to reflect and focus sunlight onto a thermal receiver. The intense CSP energy heats up the fluid (heat-transfer fluid or HFT) in the receiver to high temperatures. This heat or thermal energy is used to turn a turbine and thus generate electricity (source:). Unlike the solar energy generated by photovoltaic panels, the thermal energy contained in the fluid can be stored for later use. CSP energy also has direct industrial applications in water desalination and food processing.
Concentrated solar power also has a high energy output, which makes it suitable for large-scale electricity generation. One of the main drawbacks is its high capital and maintenance costs. CSP installations require significant upfront investments, Additionally, the technology is still relatively new, and there’s a lack of experienced professionals in the field.
Wind energy using the aerodynamic force
Wind turbines use wind to make electricity. Wind turns the propeller-like blades of a turbine around a rotor, which spins a generator, which creates electricity. into electricity using the aerodynamic force from the rotor blades, which work like an airplane wing or helicopter rotor blade. When wind flows across the blade, the air pressure on one side of the blade decreases. The difference in air pressure across the two sides of the blade creates both lift and drag. The force of the lift is stronger than the drag and this causes the rotor to spin. The rotor connects to the generator that speed up the rotation and allow for a physically smaller generator. This translation of aerodynamic force to rotation of a generator creates electricity (source ). The permanent magnets are in the generator of the largest wind turbines to reduce costs, improve reliability and reduce the need for maintenance (source: ).
Technologies to produce synthetic fuels
Synthetic fuels are liquid fuels that have the same properties as fossil fuels but are produced artificially. Synthetic fuels can be blended with fossil fuels or replace the fossil fuel in internal combustion engines in vehicles, planes or ships. For the production of synthetic fuels CO2 is captured from the atmosphere through Direct Air Capture system (see below). Burning the synthetic fuel does release CO2 back into the air. This is the CO2 that was used in the production of the synthetic fuel. As a result, there are no-net CO2 emissions. There are four types of synthetic fuels and the way they are produced makes the difference (source ).
Biomass-to-liquid produces biofuels (any fuel that is derived from biomass) such as renewable diesel/hydrotreated vegetable oil (HVO)
Power-to-liquid produces e-fuels such as e-kerosine and e-methanol
Power-to-gas produces e-methane
Power-to-gas is a technology that uses electric power to produce a gaseous fuel. The hydrogen that is produced via electrolysis is converted to methane together with carbon dioxide. For mobility if synthetic fuels are mentioned they often refer to e-fuels or electrofuels. These fuels are produced via the power-to-liquid method. First, renewable electricity is generated, which then drives an electrolyser that splits water into hydrogen and oxygen. Next, the hydrogen is mixed with carbon dioxide and turned into syngas via the reverse water gas shift (RWGS) reaction – a process that is conducted at high temperatures and driven by electricity.
There are low carbon fuels, carbon neutral fuels and zero-carbon fuels. Low carbon fuels emit less carbon than fossil fuels. Renewable diesel, biodiesel hydrogen/methanol/ammonia when produced using fossil fuels with carbon capture and storage are examples of low carbon fuels. Renewable diesel’s CO2 emissions are highly dependent on the feedstock used. Often, this results in life-cycle emissions above zero. Carbon neutral fuels are fuels that do not increase or decrease the amount of carbon in the atmosphere through their life cycle. Power-to-liquid fuels e-methanol and e-kerosine are carbon neutral fuels. They are considered carbon neutral if renewable resources are used in the production process and the carbon captured from the atmosphere is later released back into the air. Zero-carbon fuels are fuels that do not release carbon at the time of usage. For example hydrogen when produced by electrolysis and renewable electricity and ammonia when produced by renewable electricity and green hydrogen as the source are zero-carbon fuels (source ).
Carbon capture and storage
The idea behind CCS is to capture the CO2 generated by burning fossil fuels before it is released into the atmosphere. Most current CCS strategies involve the injection of CO2 deep underground. This forms a “closed loop”, where the carbon is extracted from the Earth as fossil fuels and then is returned to the Earth as CO2. There has also been considerable interest recently in using CCS technologies to remove CO2 from the atmosphere. One option is with CCS (BECCS), where biomass (like wood or grasses) removes CO2 from the air through photosynthesis. The biomass is then harvested and burned in a power plant to produce energy, with the CO2 being captured and stored. This creates what is called “negative emissions” because it takes CO2 from the atmosphere and stores it. Another negative emission option is called direct air capture (DAC), where CO2 is removed from the air using a chemical process. However, the concentration of CO2 in the air is about 300 times less than in the smokestacks of power plants or industrial plants, making it much less efficient to capture. Because of this, DAC is quite an expensive option today (source ).
Combined heat and power
Cogeneration or combined heat and power (CHP) is the use of a heat engine or power station to generate electricity and useful heat at the same time. Cogeneration is a more efficient use of fuel or heat, because otherwise-wasted heat from electricity generation is put to some productive use. This involves the concurrent production of electricity or mechanical power and useful thermal energy (heating and/or cooling) from a single source of energy (source: ). Combined heat and power (CHP) is a highly efficient process (over 80%) that captures and utilises the heat that is a by-product of the electricity generation process. The electricity could be generated from renewables as well. By generating heat and power simultaneously, CHP can reduce carbon emissions by up to 30% compared to the separate means of conventional generation via a boiler and power station. The heat generated during this process is supplied to an appropriately matched heat demand that would otherwise be met by a conventional boiler.
Digital technologies
The are several digital technologies that improve efficiency and reduce demand for heat and or electricity. For example smart technologies that monitor and manage the demand/use of heat and electricity. A smart grid is an electricity network that uses digital and other advanced technologies to monitor and manage the transport of electricity from all generation sources to meet the varying electricity demands of end users (source: ). Another technology is the Internet-of-Waste (IoT). IoT-enabled waste management and recycling significantly reduce the inefficiencies in waste logistics. From fill-level sensors to smart bins and material quality assessing sensors, the recycling industry is leveraging the internet of waste to streamline operations.
Conclusion
We have defined eleven technologies/group of technologies that help to decarbonize the world by 2050 namely: lithium-ion batteries, heat pumps, permanent magnets, electrolysis & fuel cells, photovoltaics, concentrated solar power, wind energy using aerodynamic force, technologies that produce synthetic fuels, carbon capture and storage and combined heat and power and digital technologies. But as technologies are continuously evolving because of limitations and challenges this list is not complete and will also change over time. So it is by no means a list with technologies that is fixed for the future. Companies in many sectors have a lot of low-hanging fruit when it comes to decarbonisation technologies. For further analysis on how this manifests itself in sectors, see the publication .